Tracking dispersal across a patchy landscape
- Edelsparre, A. H.
- Oct 10, 2020
- 4 min read
Historically, as well as contemporary, dispersal remains one of the most important biological processes on our planet. Throughout history, dispersal facilitated the colonization of all kinds of environments that support life on our planet. Today, biodiversity face new challenges as climate change and habitat loss from human activities assert pressures on ecosystems. Just like in the past dispersal will, in part, be the key to how (and how fast) populations can respond to these challenges. Uncovering key features of the landscape that facilitate or impede movement among habitat patches is therefore critical to understand how changes in the landscape affect dispersal at the population-level and ultimately the distribution of species and communities over time.
In our laboratory we are interested in addressing such issues experimentally. The great advantage with conducting experiments in the lab is that you get to change specific landscape features under controlled conditions and observe how an organism changes its behaviour. In our recent paper in Oikos we describe a study where we examined how flies (Drosophila melanogaster, Image 1) colonized food patches in landscapes with different distances among patches and with different patch distributions.
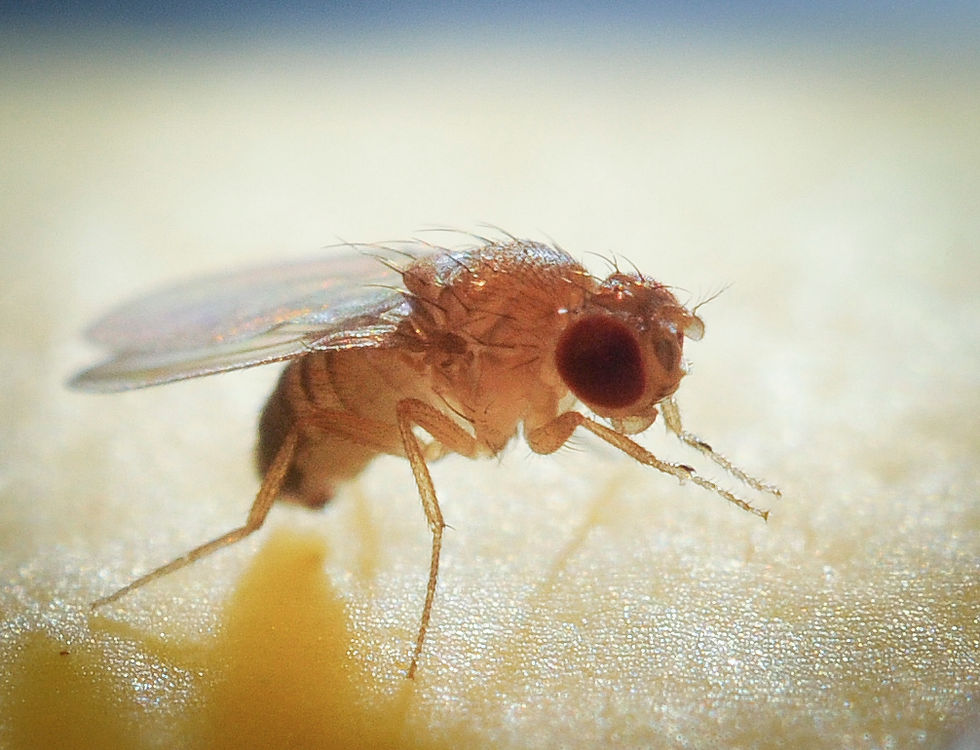
Image 1: The common fruit fly, Drosophila melanogaster (Photo credit: Ken Jones).
Image 2 and 3 show an example of one of our experimental landscapes. These landscapes consisted of 25 locations (cells) connected via clear tubes that provided access to adjacent locations. If a cell contained food it was treated as a food patch. The 25 locations were arranged in a 5 by 5 array and with a central location from which flies were allowed to disperse from. We used this general set up to run trials in landscapes with different inter-patch distances and with different patch distributions. This allowed us to examine the effect of each landscape feature on dispersal.

Image 2: Showing a cell with food (e.g. food patch) (Photo credit: Allan Edelsparre).
A second, and equally interesting question we were interested in asking is how does variation in dispersal strategy interact with the distribution and pattern of food patches in the landscape. This is an important question to ask because in many cases landscape models assume that dispersers are drawn from a random population. This can be problematic if individuals consistently differ in behaviour under some environmental conditions, but similarly under other environmental conditions. To answer this question we examined two different strains of flies known for their genetic dispersal predispositions. One of these strains (rovers) carried a version of the foraging (for) gene that is linked with greater dispersal propensity (forR). The second strain (sitters) carried a version of (for) linked with lower dispersal propensity (fors). We also examined an outbred population of flies with an unknown dispersal strategy and which represented a general population’s response to changes in the landscape.
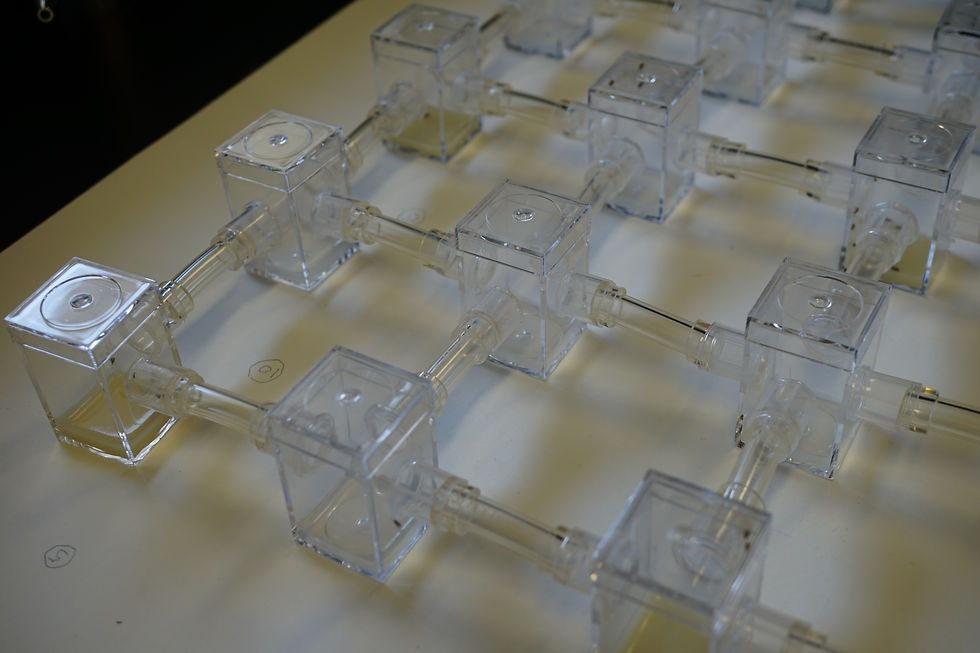
Image 3: Showing a section of the landscape with cells that are connected via a clear passageway (Photo credit: Allan Edelsparre).
Our experiments made two key findings. First, we found that inter-patch distances were extremely important for connectivity in landscapes. Even when the landscape was packed with food patches rovers (more dispersive) were limited in colonizing new food patches. The outbred population was also severely impacted by distances; only a small fraction of this population were able to colonize a new food patch when inter-patch distances were large. As you would expect, the effect of distance was even more pronounced in sitters (less dispersive) where dispersal was reduced to near zero. This highlights the importance of creating corridors that facilitate the movement of less dispersive phenotypes as well as more dispersive phenotypes. If not, this could have important implications for genetic variation in general if distances extend beyond the dispersal capacity of a critical part of a population.
The second finding our experiments made is that the distribution and number of food patches influenced flies in two strikingly different ways. For example, if the total quality of the landscape was fixed both rovers and sitters increased their colonization of new food patches as the density and number of patches increased within the landscape. However, when the total quality of the landscape was allowed to increase with the number of food patches rovers and sitters adopted very different strategies to colonize each landscape (rovers increase dispersal and sitters decrease dispersal). This is really interesting because it suggests that dispersal could result from a process that alternates between strong G x E interaction (genotypes track the environment differently) and conditions with strong environmental effects (genotypes track the environment similarly).
Ecologists often deal with complex landscapes with many possible combinations of inter-patch distances and distributions. Our study demonstrated that knowing how specific landscape features influence and interact with dispersal may provide ecologists an improved opportunity to predict how organisms are going to respond to environmental perturbations such as those imposed by habitat loss and fragmentation. An important future step will be to understand how interactions such as those elucidated in our study will affect the eco-evolutionary dynamics of dispersal in particular and population dynamics in general.
Comments